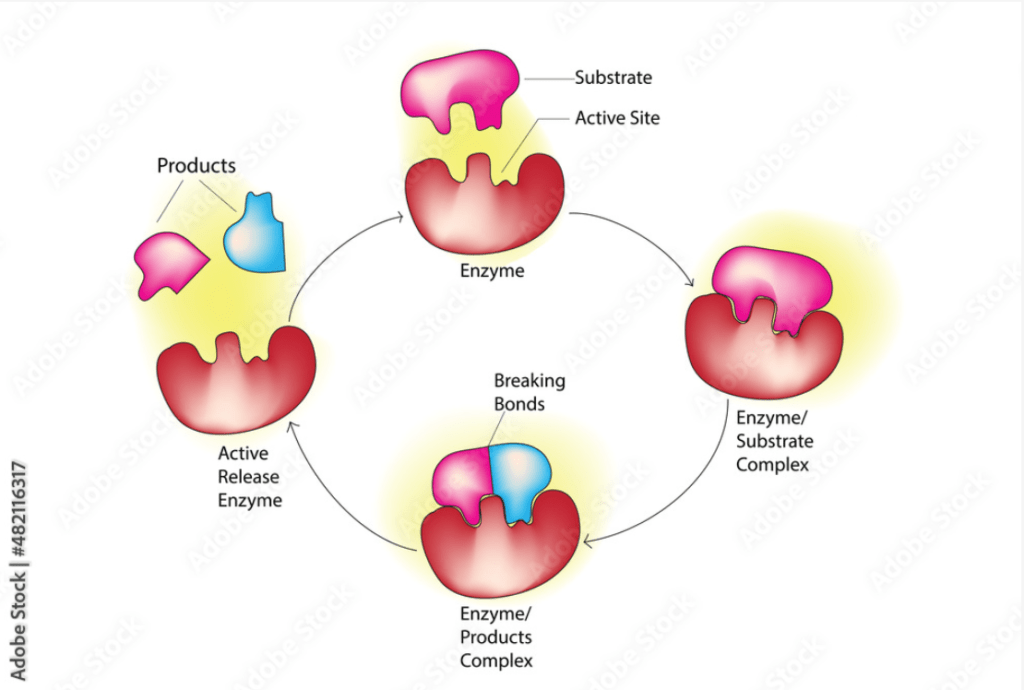
Enzymes are biological catalysts that speed up chemical reactions in living organisms. They are typically proteins, although some RNA molecules also exhibit catalytic activity and are termed ribozymes. Here’s a detailed look at the definition and nomenclature of enzymes:
Definition of Enzymes
Enzymes are molecules, primarily proteins, that facilitate and accelerate biochemical reactions without being consumed or permanently altered in the process. They achieve this by lowering the activation energy required for reactions to proceed, allowing cellular processes to occur more efficiently and under milder conditions than would otherwise be possible.
Concept of Activation Energy
Activation energy is the minimum amount of energy required for a chemical reaction to proceed. It represents the energy barrier that must be overcome for reactants to be transformed into products. Here are the key points about activation energy:
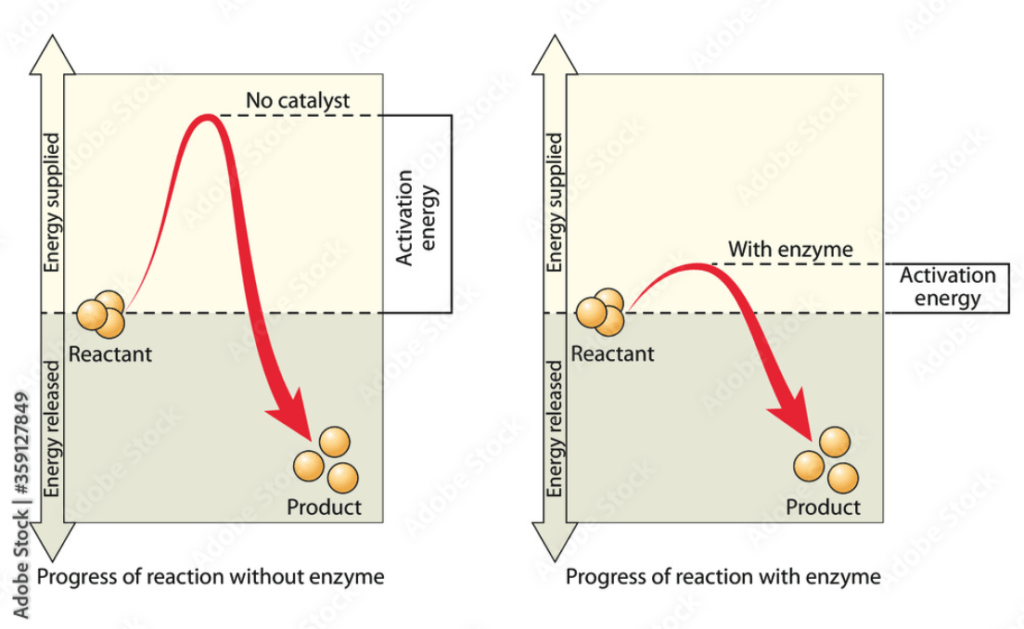
- Energy Barrier: Activation energy is like a hill that reactants need to climb before they can convert into products. Even if the overall reaction releases energy (exothermic), an initial input of energy is required to start the reaction.
- Transition State: During a reaction, molecules pass through a high-energy, unstable intermediate state known as the transition state. The activation energy is the energy difference between the reactants and this transition state.
- Effect on Reaction Rate: The higher the activation energy, the slower the reaction rate, because fewer molecules will have sufficient energy to reach the transition state. Conversely, a lower activation energy means more molecules can react, leading to a faster reaction.
- Temperature Dependence: Increasing the temperature provides more energy to the molecules, increasing the number of molecules with enough energy to overcome the activation energy barrier, thus speeding up the reaction.
- Catalysts: Catalysts are substances that lower the activation energy of a reaction without being consumed in the process. They provide an alternative reaction pathway with a lower energy barrier, increasing the reaction rate.
Example
For the decomposition of hydrogen peroxide (H₂O₂) into water (H₂O) and oxygen (O₂):
2H2O2 → 2H2O + O2
The reaction is slow at room temperature because of the high activation energy. Adding a catalyst like manganese dioxide (MnO₂) significantly lowers the activation energy, speeding up the reaction.
Enzyme-Substrate Complex
The enzyme-substrate complex is a temporary molecule formed when an enzyme binds to its substrate. This interaction is fundamental to the enzyme’s catalytic function.
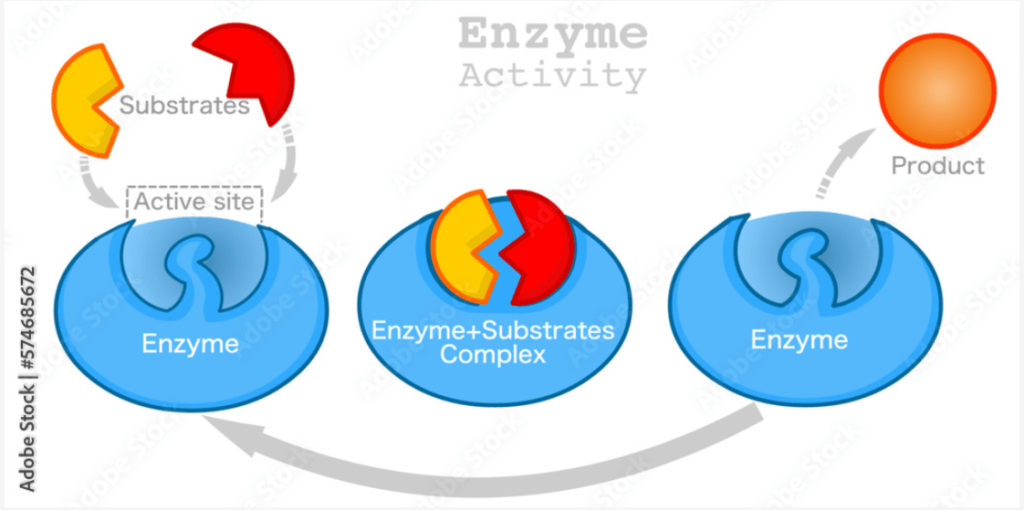
Steps in Enzyme-Substrate Interaction:
1. Binding:
- The substrate binds to the enzyme’s active site, forming the enzyme-substrate complex.
- This binding can involve hydrogen bonds, ionic bonds, hydrophobic interactions, and Van der Waals forces.
2. Formation of the Transition State:
- The enzyme stabilizes the transition state of the reaction, reducing the activation energy required for the reaction to proceed.
- This transition state is often different from both the substrate and the product.
3. Catalysis:
- The enzyme facilitates the conversion of the substrate into the product through various mechanisms such as acid-base catalysis, covalent catalysis, and metal ion catalysis.
- Acid-Base Catalysis: Involves proton transfer to stabilize transition states.
- Covalent Catalysis: Involves transient covalent bonds between catalyst and substrate.
- Metal Ion Catalysis: Utilizes metal ions to stabilize charges and participate in reactions.
4. Product Release:
- The products of the reaction are released from the enzyme, which can then bind to new substrate molecules and repeat the process.
Key Properties of Enzymes:
Catalytic Efficiency:
- Enzymes increase the rate of reactions by lowering the activation energy required for the reaction to proceed.
- They can catalyze reactions at rates that are millions of times faster than those without a catalyst.
Specificity:
- Enzymes are highly specific for their substrates (the molecules they act upon).
- This specificity is due to the unique three-dimensional structure of the enzyme, particularly at the active site where substrate binding occurs.
Regulation:
- Enzyme activity can be regulated by various means including feedback inhibition, covalent modification (e.g., phosphorylation), and by the presence of inhibitors or activators.
Sensitivity to Environmental Conditions:
- Enzyme activity is influenced by factors such as temperature, pH, and ionic strength. Extreme conditions can lead to denaturation and loss of activity.
Reversibility:
- Enzyme-catalyzed reactions are often reversible, meaning the enzyme can catalyze both the forward and reverse reactions, depending on the concentrations of substrates and products.
Nomenclature of Enzymes
Common Naming Conventions
The nomenclature of enzymes is standardized by the Enzyme Commission (EC) of the International Union of Biochemistry and Molecular Biology (IUBMB). The system classifies enzymes based on the type of reaction they catalyze. While the EC number provides a systematic way to classify enzymes, common names are often used for simplicity. These names are usually derived from the substrate they act on or the type of reaction they catalyze, often ending in “-ase.” For example:
- Lactase: An enzyme that breaks down lactose.
- Protease: An enzyme that hydrolyzes proteins.
Examples and Specificity
- Specificity: Enzymes are highly specific both in the reactions they catalyze and in their choice of substrates. This specificity is due to the precise interaction between the enzyme’s active site and the substrate.
- Example: Catalase (EC 1.11.1.6) is an enzyme that catalyzes the decomposition of hydrogen peroxide to water and oxygen.
EC Number Format
Each enzyme is assigned a unique EC number consisting of four parts, each separated by a period, indicating a specific enzyme’s subclass and sub-subclass. An EC number is written as EC X.X.X.X, where each X represents a number specifying the enzyme’s classification:
- First Number: Main class (the general type of reaction catalyzed).
- Second Number: Subclass (more specific type of reaction within the main class).
- Third Number: Sub-subclass (further specification within the subclass).
- Fourth Number: Serial number of the enzyme in its sub-subclass.
IUB Classification of Enzymes
The International Union of Biochemistry and Molecular Biology (IUBMB) classifies enzymes based on the reactions they catalyze. Enzymes are divided into six major classes, each with subclasses based on more specific reaction types. Each enzyme is assigned an Enzyme Commission (EC) number.
Main Classes of Enzymes (EC 1 to EC 7)
1. Oxidoreductases (EC 1):
Catalyze oxidation-reduction reactions, where electrons are transferred between molecules.
- Example: Dehydrogenases, oxidases.
Generalized Reaction: A + B → A’ + B’
Example Reaction: Catalyzed by Lactate Dehydrogenase
L-lactate + NAD+ → pyruvate + NADH + H+
Description: This reaction involves the oxidation of L-lactate to pyruvate with the concomitant reduction of NAD(^+) to NADH.
2. Transferases (EC 2):
Transfer functional groups (e.g., methyl, glycosyl) from one molecule to another.
- Example: Kinases, transaminases.
Generalized Reaction: A + BX → AX + B
Example Reaction: Catalyzed by Hexokinase
Glucose + ATP → glucose-6-phosphate + ADP
Description: This reaction involves the transfer of a phosphate group from ATP to glucose, forming glucose-6-phosphate.
3. Hydrolases (EC 3):
Catalyze the hydrolysis of various bonds (e.g., ester, glycosidic, peptide).
- Example: Proteases, lipases.
Generalized Reaction: AB + H2O → A + B
Example Reaction: Catalyzed by Lipase
Triacylglycerol + 3H2O → glycerol + 3 fatty acids
Description: This reaction involves the hydrolysis of triacylglycerols into glycerol and free fatty acids.
4. Lyases (EC 4):
Add groups to or remove groups from double-bonded substrates, often forming or breaking double bonds.
- Example: Decarboxylases, synthases.
Generalized Reaction: AB → A + B
Example Reaction: Catalyzed by Pyruvate Decarboxylase
Pyruvate → acetaldehyde + CO2
Description: This reaction involves the non-hydrolytic removal of a carboxyl group from pyruvate, resulting in the formation of acetaldehyde and carbon dioxide.
5. Isomerases (EC 5):
Catalyze the rearrangement of atoms within a molecule, resulting in isomers.
- Example: Isomerases, epimerases.
Generalized Reaction: A → B
Example Reaction: Catalyzed by Phosphoglucoisomerase
Glucose-6-phosphate → fructose-6-phosphate
Description: This reaction involves the isomerization of glucose-6-phosphate to fructose-6-phosphate.
6. Ligases (EC 6):
Catalyze the joining of two molecules coupled with the hydrolysis of a diphosphate bond in ATP or a similar triphosphate.
- Example: DNA ligase, synthetases.
Generalized Reaction: A + B + ATP → AB + ADP + Pi
Example Reaction: Catalyzed by DNA Ligase
(DNA strand)n + (DNA strand)m + ATP → (DNA strand)n+m + AMP + PPi
Description: This reaction involves the joining of two DNA strands by forming a phosphodiester bond between them, with the concomitant hydrolysis of ATP to AMP and pyrophosphate (PPi).
7. Translocases (EC 7):
Translocases are a class of enzymes that catalyze the movement of ions or molecules across membranes or their separation within membranes. Translocases are essential for various physiological processes, including nutrient uptake, waste removal, ion balance, and maintaining membrane potential. Their ability to move substances across membranes using energy from ATP or other sources makes them critical components of cellular function. This class was added to the Enzyme Commission (EC) system to accommodate these important biological functions.
- Example: Synthases.
Generalized Reaction: Ain + energy → Aout
Here, Ain represents the molecule or ion on one side of the membrane, and Aout represents the molecule or ion translocated to the other side of the membrane. The energy needed for this translocation can come from various sources, such as ATP hydrolysis.
Example Reaction
- ATP-Binding Cassette (ABC) Transporters: Catalyzed by ABC Transporters
Substratein + ATP → Substrateout + ADP + Pi
Description: ABC transporters use the energy from ATP hydrolysis to transport substrates across cellular membranes. - Proton Pump: Catalyzed by H+-ATPase (ATP Synthase)
H+in + ATP → H+out + ADP + Pi
Description: H+-ATPases pump protons (H+) across membranes using energy from ATP hydrolysis, crucial for maintaining pH gradients and membrane potentials. - Sodium-Potassium Pump Na+/K+-ATPase: Catalyzed by Na+/K+-ATPase
Na+in + 2k +out + ATP → 3Na+out + 2K+in + ADP + Pi
Description: This pump uses ATP hydrolysis to transport sodium ions out of the cell and potassium ions into the cell, crucial for maintaining cellular ion balance and membrane potential.